Quantum Computing’s Promise: Solving Previously Unsolvable Problems
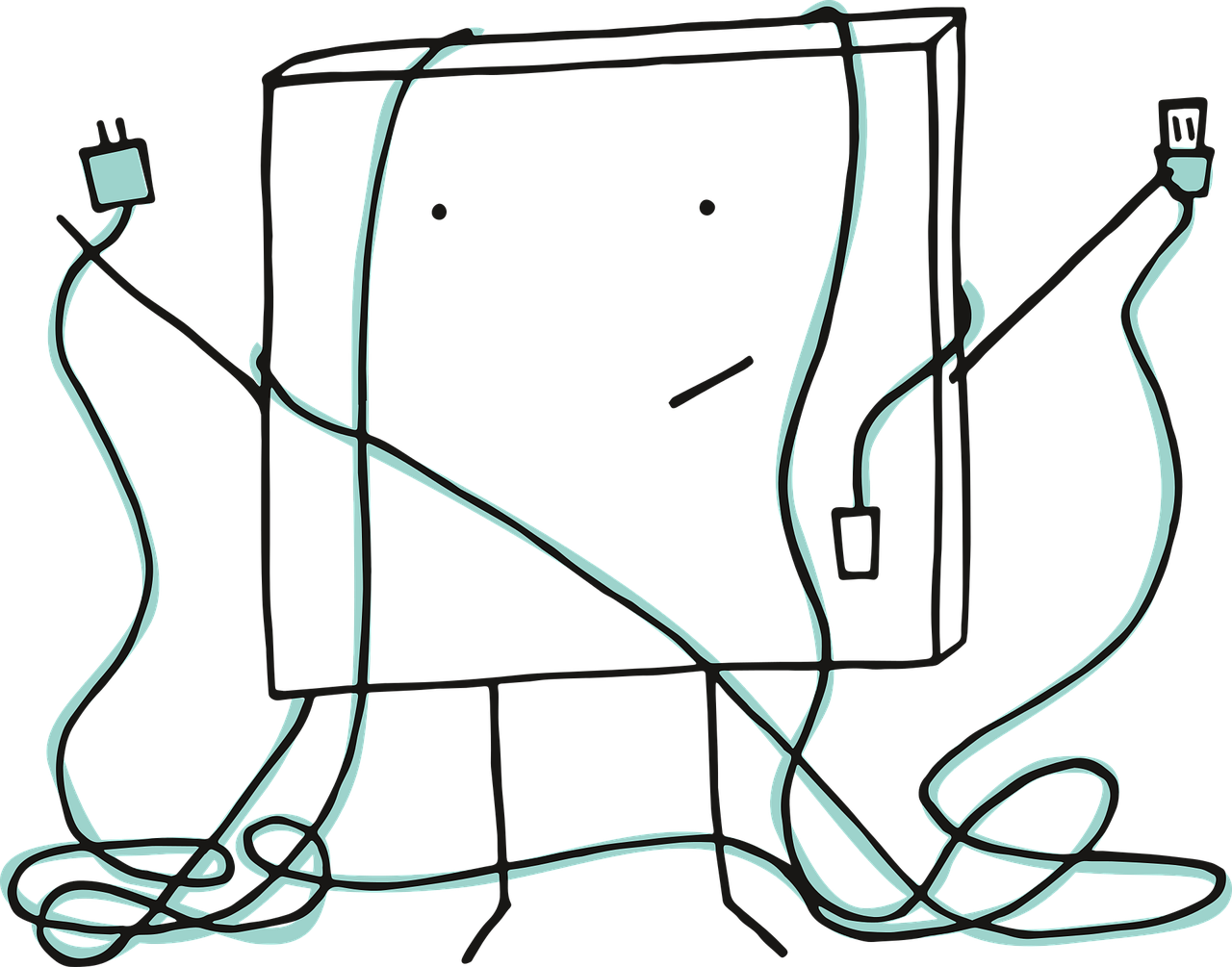
In recent years, quantum computing has moved from the realm of theoretical physics into the forefront of technological innovation. Promising to revolutionize industries by solving problems that have long been considered unsolvable by classical computers, quantum computing holds immense potential across fields as diverse as cryptography, drug discovery, artificial intelligence (AI), and materials science. At its core, quantum computing leverages the principles of quantum mechanics—such as superposition and entanglement—to perform calculations far beyond the capabilities of traditional computing systems.
This article explores the transformative power of quantum computing, the types of previously unsolvable problems it can address, and the implications for various industries. By unlocking new dimensions of computational power, quantum computing could fundamentally change how we approach problem-solving in science, technology, and beyond.
Introduction: The Dawn of Quantum Computing
Classical computers, the digital machines that have powered the world for decades, operate on bits that exist in one of two states—either 0 or 1. These binary systems form the foundation of all modern computing, allowing for the manipulation of data to solve a wide range of problems. However, there are certain complex problems, particularly in fields like cryptography and optimization, that classical computers struggle to solve efficiently. These problems often involve an exponential number of possible solutions, requiring more computational power and time than traditional systems can provide.
Quantum computing introduces a new paradigm. Unlike classical bits, quantum bits—or qubits—can exist in multiple states simultaneously thanks to the principle of superposition. This means that a quantum computer can process a vast number of possibilities at once. Moreover, qubits can become entangled, allowing for instantaneous communication between them, further enhancing the computational potential. By exploiting these quantum properties, quantum computers have the potential to solve problems that are currently intractable for classical computers.
As we explore the promise of quantum computing, it is important to recognize that we are still in the early stages of its development. However, even in its infancy, quantum computing has already demonstrated the potential to transform industries and solve problems previously deemed unsolvable.
Quantum Mechanics at the Core of Quantum Computing
Superposition and Parallelism
At the heart of quantum computing lies the principle of superposition. In a classical computer, each bit represents a single state—either a 0 or a 1—at any given time. In contrast, a qubit can represent both 0 and 1 simultaneously, thanks to superposition. This allows quantum computers to perform many calculations in parallel, exponentially increasing the amount of data they can process.
For example, if you have two qubits, they can represent four possible states simultaneously: 00, 01, 10, and 11. With three qubits, that number rises to eight possible states, and with every additional qubit, the number of possible states doubles. This exponential growth enables quantum computers to explore multiple potential solutions to a problem at once, making them incredibly powerful for tasks that involve large datasets or complex computations.
Entanglement and Quantum Speedup
Another key feature of quantum computing is entanglement, a phenomenon in which qubits become linked, such that the state of one qubit instantly affects the state of another, no matter how far apart they are. Entanglement allows for instantaneous communication between qubits, enabling faster information processing and the potential for quantum speedup.
Quantum speedup refers to the ability of a quantum computer to solve certain problems significantly faster than a classical computer. For example, problems that involve searching large databases or factoring large numbers could be solved exponentially faster on a quantum computer compared to the best-known classical algorithms.
Previously Unsolvable Problems and Quantum Computing
Quantum computing has the potential to tackle a wide range of problems that are currently beyond the reach of classical computers. These include problems in cryptography, materials science, drug discovery, and optimization, all of which could benefit from the unique computational power of quantum systems.
Cryptography: Breaking and Building the Future of Security
One of the most widely discussed applications of quantum computing is its potential to break modern cryptographic systems. Most encryption methods, such as RSA (Rivest–Shamir–Adleman) encryption, rely on the difficulty of factoring large prime numbers, a task that classical computers struggle with when the numbers involved are sufficiently large.
Quantum computers, however, could use algorithms like Shor’s algorithm to factor these large numbers exponentially faster than classical computers, potentially rendering current encryption methods obsolete. This poses a significant threat to digital security, as encrypted data, including financial transactions, personal information, and government communications, could be decrypted in a fraction of the time it would take with classical systems.
While this may sound alarming, quantum computing also offers the potential to build new, quantum-resistant encryption methods. Quantum key distribution (QKD), for example, allows for the secure transmission of encryption keys using the principles of quantum mechanics. Any attempt to intercept or measure the quantum key would disturb the system, alerting both parties to the presence of an eavesdropper and ensuring the integrity of the communication.
Drug Discovery and Molecular Simulation
Drug discovery is another field that stands to benefit immensely from quantum computing. The process of discovering new drugs involves simulating molecular interactions, which can be computationally intensive due to the vast number of variables involved. Classical computers struggle to accurately simulate even moderately complex molecules because of the exponential growth in possible interactions as the number of atoms increases.
Quantum computers, however, can model these interactions much more efficiently. By leveraging quantum properties like superposition and entanglement, quantum systems can simulate the behavior of molecules and proteins at the quantum level, providing insights that are impossible to achieve with classical systems. This could lead to the discovery of new drugs, treatments for diseases, and a deeper understanding of complex biological processes.
For example, quantum simulations could help researchers design drugs that more precisely target specific proteins or receptors, reducing the risk of side effects and improving efficacy. In the future, quantum computing could significantly accelerate the drug development process, saving time, money, and lives.
Optimization Problems: Logistics, Finance, and Beyond
Optimization problems are ubiquitous across industries, from logistics and transportation to finance and manufacturing. These problems involve finding the best possible solution from a vast set of possible solutions, such as determining the most efficient delivery route for a fleet of trucks or optimizing investment portfolios.
Classical computers struggle with optimization problems because the number of possible solutions often grows exponentially with the size of the problem. For example, the traveling salesman problem, which involves finding the shortest route through a set of cities, becomes computationally intractable as the number of cities increases.
Quantum computers, however, can approach optimization problems from a different angle. By exploring multiple potential solutions simultaneously, quantum systems can identify optimal solutions much more quickly than classical algorithms. This has significant implications for industries that rely on complex decision-making processes.
For example, in finance, quantum computing could be used to optimize investment strategies by analyzing vast amounts of market data and simulating different portfolio configurations. In logistics, quantum systems could help companies optimize supply chains, reducing costs and improving efficiency.
Artificial Intelligence and Machine Learning
Artificial intelligence (AI) and machine learning (ML) are fields that have already transformed industries ranging from healthcare to entertainment. However, current AI and ML models are often limited by the computational power of classical systems. Training large neural networks, for example, can require vast amounts of data and processing power, making it difficult to scale AI applications effectively.
Quantum computing could dramatically accelerate the training process for AI models by enabling faster data processing and more efficient optimization algorithms. Quantum systems could also improve the accuracy of AI models by exploring larger solution spaces and identifying patterns that classical systems might miss.
Quantum machine learning (QML) is an emerging field that combines quantum computing with machine learning techniques. Early research suggests that QML algorithms could outperform classical algorithms in certain tasks, such as data classification and pattern recognition. As quantum hardware continues to evolve, QML could unlock new levels of performance and efficiency in AI applications.
Materials Science and Quantum Chemistry
Materials science is another field that stands to benefit from quantum computing. The development of new materials often requires simulating the behavior of atoms and electrons at the quantum level, a task that is computationally intensive for classical systems. Quantum computers, however, are naturally suited to this type of simulation because they operate on the same quantum principles as the materials being studied.
For example, quantum computers could be used to simulate the properties of new superconductors, enabling the development of materials with zero electrical resistance. This could lead to breakthroughs in energy transmission, transportation, and electronics.
Quantum chemistry, a subset of materials science, also stands to benefit from quantum computing. By simulating chemical reactions at the quantum level, quantum computers could provide new insights into how molecules interact, leading to the development of more efficient catalysts, renewable energy sources, and advanced materials.
Challenges and the Path Forward for Quantum Computing
Despite its immense promise, quantum computing is still in its early stages. Current quantum systems, known as noisy intermediate-scale quantum (NISQ) devices, are prone to errors and are limited in the number of qubits they can support. Building a fully scalable, error-corrected quantum computer remains one of the biggest challenges in the field.
Error Correction and Decoherence
One of the main challenges in developing practical quantum computers is overcoming decoherence, a phenomenon in which quantum states lose their coherence due to environmental noise.
This leads to errors in quantum calculations, which must be corrected for quantum computers to function reliably.
Quantum error correction is a field of research dedicated to developing methods for detecting and correcting errors in quantum systems. While progress has been made, creating fault-tolerant quantum computers that can scale to solve real-world problems remains a significant hurdle.
Hardware and Scalability
Building quantum computers with large numbers of stable qubits is another challenge. Current quantum systems are limited in the number of qubits they can support, and maintaining qubit stability over time is difficult. Advances in quantum hardware, such as more stable qubits and better quantum gates, will be essential for scaling quantum computers to tackle complex, real-world problems.
The Future of Quantum Computing: Unlocking New Possibilities
Quantum computing holds the promise of solving problems that are currently unsolvable by classical computers, from breaking modern cryptography to revolutionizing drug discovery and materials science. While significant challenges remain, the potential impact of quantum computing on industries ranging from finance to healthcare is undeniable.
As quantum hardware and algorithms continue to evolve, we can expect quantum computing to unlock new possibilities, driving breakthroughs in science, technology, and industry. The future of problem-solving lies at the intersection of quantum mechanics and computing, and as we move closer to realizing the full potential of quantum systems, the world of previously unsolvable problems may soon become a thing of the past.
FAQs
What makes quantum computing different from classical computing?
Quantum computing uses qubits that can exist in multiple states simultaneously, allowing it to process vast amounts of information in parallel. Classical computers, on the other hand, use bits that are limited to either 0 or 1.
What types of problems can quantum computers solve that classical computers cannot?
Quantum computers can tackle problems involving large-scale optimization, molecular simulation, and cryptography that are currently unsolvable or inefficient to solve with classical computers.
What is superposition in quantum computing?
Superposition is the principle that allows qubits in a quantum computer to represent both 0 and 1 at the same time, enabling the system to process multiple possibilities simultaneously.
How could quantum computing impact cryptography?
Quantum computers could potentially break current encryption methods by solving complex mathematical problems, such as factoring large numbers, much faster than classical computers.
What industries will benefit the most from quantum computing?
Industries such as finance, healthcare, materials science, and logistics stand to benefit the most, as quantum computing can solve optimization problems, improve drug discovery, and simulate new materials at the quantum level.
What are the main challenges facing quantum computing?
The primary challenges include overcoming decoherence, scaling quantum hardware, and developing error-correction techniques to build fault-tolerant quantum computers.